Potassium niobate (KNbO3; KN) is a well-known ferroelectric material with a perovskite structure, discovered in 1949. There are reports of its physical properties, crystal growth, crystal structure, and other characteristics [1]. In addition, recently these crystals were found to have a high electromechanical coupling coefficient for surface acoustic waves (SAWs), which makes potassium niobate a promising material for next-generation elastic wave elements [2]. KN also has become a very promising candidate as a lead-free piezoelectric material since it was found that the coupling factors in the bulk acoustic wave (BAW) have very high values at optimum cuts [3]. This crystal has thus drawn much attention as an environmentally friendly material useable in SAW and BAW devices.
Potassium niobate is an incongruent melting compound in which KNbO3 solid phase cannot coexist in equilibrium with liquid phase. Therefore, these crystals cannot be grown by the Czochralski (CZ) method used for lithium niobate and lithium tantalate. Instead, TSSG (Top-Seeded Solution Growth) using a liquid phase of KNbO3 with excess addition of K2O, or the Bridgman method are employed. However, these methods have problems such as contamination of the crystal with flux, and cannot be used for mass production of large crystals. In addition, potassium niobate transitions from cubic crystals to tetragonal crystals at 435 °C, and to orthorhombic crystals at 225 °C. Because of these two phase transitions, single-domain processing after cooling is not easy, and multiple operations are required. Cracks and other problems occur in single-domain processing. As a result, single-domain processing of asgrown bulk crystals is presently difficult, even though large crystals could be grown at low cost. Thus, there are a number of problems to be solved for the growth of large KNbO3 crystals.
There is another melt growth method in addition to CZ and TSSG, namely, fiber growth. In particular, the main methods of growing oxide crystal fibers are the laser heated pedestal growth (LHPG) method of Feigelson [4] and the micro-Czochralski method developed by Ohnishi and Yao [5]. Pulling-down offers better crystal quality and other characteristics than pulling-up, and thus the micro-pulling-down (μ-PD) method was developed. Many oxide crystal fibers have been grown by this method [6]. Chani et al. first reported the growth of potassium niobate crystal fibers from various liquid compositions by the μ-PD method [7]. Their study is noteworthy because it showed the possibility of growing potassium niobate single-phase fibers from a liquid with stoichiometric composition. However, the report does not include growth conditions and other details. It is very important to clear the growth conditions of KN fiber crystals from KN liquid by μ-PD method.
On the other hands, the cutting loss for the manufacturing devices is normally up to 60% of the initial weight of grown crystals by CZ method, and thus it may be remarkably useful to develop new method to grow crystals with rectangular plate with similar to the width and thickness of devices for the reduction of production cost. There also is a serious challenge for crystal growers to develop so-called shaped growth techniques offering ready-to use crystals with form similar to the devices dimensions.
Therefore, we have been investigating the growth of KN plate crystals with rectangular shape, similar to the device dimensions, from the melt with a stoichiometric composition. In this paper, we describe growth of plate-shaped KN crystal form by the μ-PD method from several liquid composition including a stoichiometric composition, and also characterization of grown plate KN crystals.
The μ-PD furnace used in this study is shown in Fig.1, and the platinum crucible with a rectangular nozzle at the bottom is shown in Fig. 2. We used a cylindrical platinum crucible with rectangular nozzle (5mm×1mm, height 2mm and 0.4 mm hole at a center), stabilizing the solid-liquid interface during the growth in order to keep the crystal shape uniformly. The growth furnace is of the resistance heating type. A voltage is applied to heat the platinum crucible (Fig. 2). KN crystal powder was used as the starting materials. The staring materials were melted in a crucible. A seed crystal was brought into contact with the melt drop at the nozzle and was then pulled down. The grown plate crystal was about 35mm long, about 1.5 mm thickness and about 4 mm width; it was colorless and striation was often observed. K4Nb6O17 phase in grown crystals was also detected as the second phase in a high-temperature gradient. For a lower temperature gradient, a single-phase KN plate crystal was obtained as shown in Fig.3. Plate shaped KN crystal has been successfully grown form a stoichiometric melt.
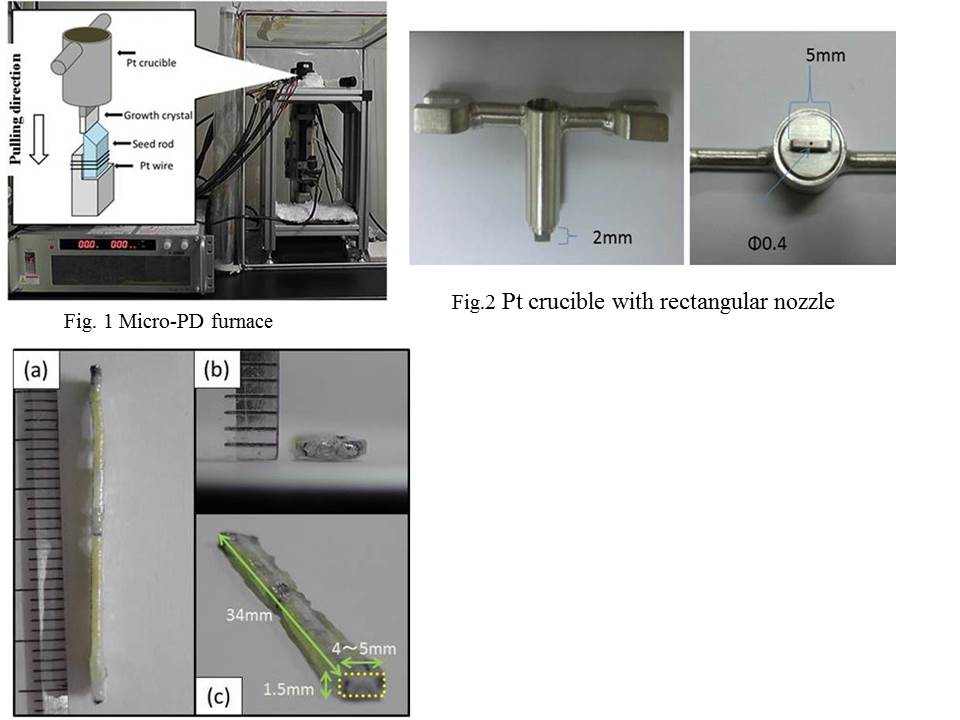
Fig.3 Grown plate KN crystal
According to the phase diagram of KN, potassium niobate should not deposit from a stoichiometric melt. The decomposition temperature of potassium niobate is, however, very close to the temperature at which all of its components melt, and thus it may be considered that potassium niobate may grow as a primary crystal from KN melt under strong supercooling in a lower temperature gradient. Large super-cooling may be absolutely necessary for this crystal growth; in the case of the μ-PD method, the degree of supercooling can be set easily higher than in the CZ method and other conventional methods. There-fore, it appears possible that single-phase potassium niobate plate crystals could be grown from a stoi-chiometric melt using potassium niobate crystals as seed crystals by this method. This single-phase KN plate crystal also showed clear periodical striations. This periodic striations may be due to the periodical change of growth rate.
The temperature and frequency dependences of the permittivity and dielectric loss (during tempera-ture descent) are also measured. At temperatures below about 400°C, there is no variation in the measured temperature range, and tanδ is smaller than 0.1. When the temperature exceeds 400 °C, tanδ increases, and dielectric dispersion occurs. In addition, sharp increases in permittivity during temperature rise at 225 and 430 °C was observed, indicating phase transitions. The phase transition temperatures of KN crystals grown by Matthias and Remeika using the flux method [8] were 224 and 434 °C, respec-tively, which are close to the results of this study. The dielectric loss too is close to that of other bulk crystals.
In conclusions, the results of this study are summarized as follows.
(1) Single-phase KN plate crystal was successfully grown from KN liquid phase by the μ-PD method. Providing a lower temperature gradient and the large supercooling beneath the nozzle is necessary for reproducible crystal growth.
(2) The phase transition temperatures of the grown KN plate crystals agreed well with previously report-ed data, and sharp transitions were observed.
(3) In addition, domains structure in grown crystal and other issues of domain control will be also dis-cussed.
Reference
1. Matthias BT, Remeika JP. Phys Rev 1949;76:1886.
2. Yamanouchi et al., Electron Lett 1997;33:193–194.
3. Nakamuraet al., IEEE Trans Ultrason Ferroelect Freq Control 2000;47:750–755.
4. Feigelson RS. J Crystal Growth 1986;79:669–680.
5. Ohnishi N, Yao T., Jpn J Appl Phys 1989;28:L278–280.
6. Yoon DH, Fukuda T., J. Crystal Growth 1994;144:201–206.
7. Chani VI et al., Crystal Res Tech 1999;34:519–525.
8. Matthias BT, Remeika JP. Phys Rev 1951;82:727–729. |